Washington, DC
January 12, 2007Source:
January
2007 issue of USDA's Agricultural Research magazine.

Molecular biologist James
Giovannoni examines high-lycopene tomatoes from
HIGH-PIGMENT 1 mutant varieties.
(D652-1)
|
In 1968, genomics breakthroughs
at ARS’s
U.S. Plant, Soil, and Nutrition Laboratory (PSNL) in Ithaca, New
York, earned the U.S. Department of Agriculture its only Nobel
Prize to date. Biochemist Robert Holley received the award for
being part of the team that first determined the structure and
nucleotide sequence of transfer RNA.
Today, PSNL scientists are
building on Holley’s legacy, applying genomics and related
sciences such as proteomics and molecular genetics to improve
the nutritional value of leading crops. The ARS researchers,
whose labs are located on Cornell University’s main campus, are
also out to boost crop plants’ resistance to disease and their
tolerance to soils lacking nutrients or containing toxic amounts
of metals. And two PSNL scientists are now part of major efforts
to map and sequence the genomes of tomato and maize.
Number Crunchers
A pivotal moment in PSNL’s
genomics research occurred 5 years ago, when David J. Schneider
was hired as one of ARS’s first computational biologists—experts
at integrating computer science with biological research. He and
molecular biologist Samuel W. Cartinhour have since helped make
computational and molecular biology critical components of the
lab’s work.

Molecular biologist Samuel
Cartinhour (left) and computational biologist David
Schneider examine a molecular model of a protein
involved in iron uptake by bacteria. Iron availability
can regulate interactions between plants and both
pathogenic and beneficial bacteria.
(D649-1)
|
“We are combining computational
and bench-based biological research to help solve complex
problems in agriculture,” says Schneider.
Schneider and Cartinhour apply
this interdisciplinary approach to their research on plant
diseases. Says Schneider, “We’re studying disease development
from the pathogen’s perspective.”
They’re using the bacterial
pathogen Pseudomonas syringae DC3000 as a model system
for studying virulence-related genes and pathways. “We’re
relying on statistical physics, computer science, and
complex-systems theory to identify regions in a pathogen’s
genome that help regulate gene expression,” says Cartinhour.
“We’re showing that there’s a real role for number-crunching in
genomics.”

ARS molecular biologist Li Li
(left) and Li-Wei Chiu, a Cornell University graduate
student, isolate novel genes from cauliflower to improve
crop nutritional value.
(D651-1)
|
Tomato’s Genes
Among other projects at Ithaca
is one led by molecular biologists James J. Giovannoni and Li
Li, who are using tomato and cauliflower as models for improving
crops’ nutritional qualities.
Five years ago, Giovannoni and
colleagues reported the discovery of RIN, a tomato gene
that regulates ethylene, a plant hormone that stimulates
ripening. This landmark finding raised the possibility of both
growing better tasting tomatoes that meet commercial shelf-life
needs and genetically manipulating ripening in other fruits,
such as melon and strawberry.
Recently, Giovannoni and
colleagues cloned tomato’s green-ripe (GR) gene, which
inhibits the plant’s ripening responses to ethylene. The gene
greatly affects fruit development while exerting minimal
influence on other plant tissues.
“This may help control
ethylene’s effects on ripening—and bring about longer shelf-life
and better quality—while retaining ethylene’s desirable effects,
such as disease resistance, on other plant tissues,” he says.
“It makes it possible to control ripening in fruit while
maintaining normal plant vigor.”

Plant pathologist Stewart Gray
prepares to microinject a virus into an aphid to
determine which insect tissues are not able to recognize
the virus, thereby preventing the aphid from
transmitting the virus.
(D650-1)
|
Giovannoni has also helped
discover two genes that regulate fruit’s response to light, and
he’s found that these genes—LeCOP1LIKE and
HIGH-PIGMENT 1—can be manipulated to alter fruit quality
and nutritional value.
Today, his team is using
microarray, or gene-chip, technology, which enables quick
examination of thousands of genes in a single experiment. One
significant study showed how microarrays can help characterize
gene expression in tomato-related fruit species, such as pepper
and eggplant, for which genomic resources are either currently
unavailable or limited.
The fruits studied are part of
the plant family Solanaceae, which—with more than 3,000
members—is the most important vegetable family. “We showed that
tomato microarrays can be used to characterize gene expression
in four of the most important Solanaceae crop species,” says
Giovannoni.
Giovannoni is also contributing
to the Tomato Sequencing Project. Undertaken by a consortium
involving scientists from 10 countries, this effort is part of
an even larger initiative: The International Solanaceae Genome
Project: Systems Approach to Diversity and Adaptation.
Cauliflower and
Beta-Carotene
Meanwhile, Li is using
cauliflower as a model system to identify genes and define
molecular mechanisms regulating the content, quality, and
availability of nutrients in plant-based foods.

Plant geneticist Edward S.
Buckler uses high-throughput robotics to efficiently
sample the DNA variation of thousands of genes in maize.
(K11756-1)
|
She’s focusing on carotenoids, the fruit-and-vegetable compounds
that the body converts into essential vitamins and uses as
antioxidants for cancer prevention. She’s using a cauliflower
gene, dubbed “Or” for the color orange, to induce
accumulation of high levels of beta-carotene in food crops.
The human body uses
beta-carotene, the carotenoid that gives carrots their color, to
make vitamin A. “Our work is important, as vitamin A deficiency
has been reported to affect some 250 million children
worldwide,” says Li.
She says the Or gene
promotes high beta-carotene accumulation in various tissues in
the cauliflower plant that normally don’t have carotenoids. “It
can help us understand how carotenoid synthesis and accumulation
are regulated in plants and in turn can help us better
understand the health benefits of carotenoids.”
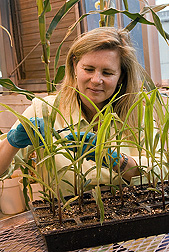
Computational biologist Doreen
Ware harvests maize tissue for RNA isolation.
(D671-1)
|
The Maize Genome
PSNL’s genomics work includes
development of statistical and genetic tools for identifying
natural variation in agronomically important traits in maize.
Scientists are also contributing to the genome sequencing of
maize.
Plant geneticist Edward S.
Buckler is working with ARS plant geneticists Michael McMullen
in the Plant Genetics Research Unit at Columbia, Missouri, and
Jim Holland in the Plant Science Research Unit at Raleigh, North
Carolina, and Stephen Kresovich, director of Cornell’s Institute
for Genomic Diversity.
“We’re analyzing many related
families of corn as well as unrelated, genetically diverse corn
lines,” says Buckler. “We are looking for genes and novel
alleles, or variations, that control maize’s complex
quantitative traits, such as yield, flower development, and seed
quality.
“By using this approach, the
best genetic variants can be discovered, and their position
within the genome can be resolved to a single gene,” he adds.
“This can help us identify genes that can spur a wide array of
traits, such as kernel quality, nutritional content, and
tolerance of soil-related stresses.”
PSNL computational biologist
Doreen H. Ware, who works at the nonprofit Cold Spring Harbor
Laboratory in New York, is contributing genome annotation and
bioinformatic tools to the sequencing of the maize genome. This
project is being funded by the National Science Foundation
(NSF), USDA, and the U.S. Department of Energy.
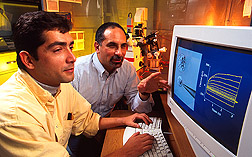
Progress in phytoremediation
studies is limited by the complexity of metal transport
and tolerance mechanisms. Here, Cornell University
research associate Miguel Pineros (left) and ARS plant
physiologist Leon Kochian study some of these mechanisms
in corn.
(K8782-1)
|
Tolerating Bad Soil
Plant physiologist Leon V.
Kochian, research leader of PSNL’s Plant, Soil, and Nutrition
Research Unit, is using similar genomic and molecular genetic
techniques in work—partially funded by NSF—to improve crop-plant
cultivation on marginal, and even highly acidic, soils that
limit crop production worldwide.
With the genomic tools used on
maize and rice—some of which are being developed by Buckler and
Ware—Kochian and his team have identified genes and associated
mechanisms that help plants tolerate soil acidity and toxic
metals.
“We’ve zeroed in on aluminum
tolerance in maize and sorghum,” Kochian says. “Aluminum is what
limits root-system growth in acid soils. These crops are ideal
for this project because in sorghum, aluminum tolerance is a
simple trait, while in maize, the tolerance is complex.”
Kochian’s group and researchers
at Brazil’s EMBRAPA Maize and Sorghum Research Center have
cloned Alt SB, the major sorghum aluminum-tolerance
gene. And recently, he and colleagues confirmed the importance
of a gene called AtALMT1 to aluminum tolerance in
Arabidopsis. They also found that a second, still
unidentified, gene plays a major role in that plant’s aluminum
tolerance in acidic soil.
Targeting Insect
Vectors
In PSNL’s Plant Protection
Research Unit, plant pathologist Stewart Gray is using genomics
to find genes that regulate plant virus transmission by insect
vectors.
He’s focused on how aphids
transmit barley yellow dwarf and potato leafroll, the most
economically important viruses of wheat, barley, oats, and
potatoes worldwide. “We want to identify both the virus genes
and the aphid genes that regulate transmission of the virus
between insect and host,” says Gray.
Recently, Gray identified and
characterized the two virus genes that regulate how a virus
moves through its aphid vector. Now his group is out to identify
the corresponding genes in aphids regulating the insects’
interaction with the virus.
Also, Gray and Iowa State
University scientists are determining the complete nucleotide
sequences of up to 100 biologically important barley yellow
dwarf and cereal yellow dwarf isolates from around the world.
His lab is also part of a scientific consortium that’s
sequencing the aphid genome.
Meanwhile, Holley’s legacy will
continue on into the lab’s future. Planning is under way to
transform the PSNL into the Robert W. Holley Center for
Agriculture and Health. This center would house all PSNL
scientists within a new, $40 million building.
By
Luis
Pons, Agricultural Research Service Information Staff.
This research is part of
Plant Genetic Resources, Genomics, and Genetic Improvement
(#301), Plant Biological and Molecular Processes (#302), Plant
Diseases (#303), Crop Protection and Quarantine (#304), and Crop
Production (#305), five ARS National Programs described on the
World Wide Web at
www.nps.ars.usda.gov.
To reach scientists
mentioned in this article, contact
Luis Pons, USDA-ARS
Information
Staff, 5601 Sunnyside Ave., Beltsville, MD 20705-5129; phone
(301) 504-1628, fax (301) 504-1486.
"Improving
Crop Plants Through Genomics" was published in the
January
2007 issue of Agricultural Research magazine. |